How Does Radiation Really Affect the Human Body?
Explore the science behind ionizing and non-ionizing radiation. Learn about short-term and long-term health effects, dose impacts, medical uses, and safety guidelines based on current research.
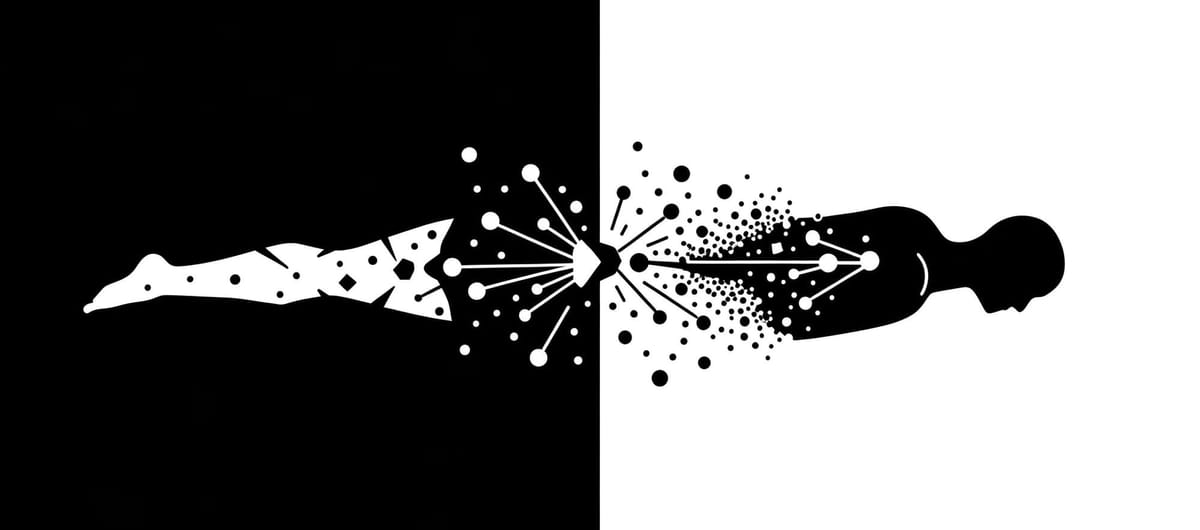
Ever wondered about the invisible forces around us? Radiation, often shrouded in mystery or depicted dramatically in fiction, is constantly present in our lives. But what does science say about its effects on our health? Let's break down the key findings from recent research.
What Exactly is Radiation?
Think of radiation as energy traveling through space. It comes in two primary flavors:
- Ionizing Radiation: High-energy stuff like X-rays, gamma rays, and particles from radioactive materials. This type has enough power to knock electrons off atoms, potentially damaging our cells and DNA.
- Non-Ionizing Radiation: Lower-energy forms like radio waves, microwaves, and even visible light. While it can't ionize atoms, it can have other effects, like heating tissue.
We encounter radiation daily from natural sources (soil, water, cosmic rays, radon gas) and human-made ones (medical scans, cancer treatments, industrial uses). Understanding its effects helps us weigh the benefits (like life-saving medical imaging) against the risks and set safety standards.
How Ionizing Radiation Impacts Our Cells
When ionizing radiation hits our cells, it can cause trouble in two ways:
- Direct Hit: The radiation directly strikes and damages our DNA.
- Indirect Hit: Radiation interacts with water molecules in our cells, creating reactive molecules (free radicals) that then damage DNA and other cell parts.
Our cells are resilient and can often repair minor damage. But if the repair fails, mutations can occur, potentially leading to cancer down the line. If the damage is too severe, the cell might die. Widespread cell death in an organ can cause serious immediate problems.
Short-Term vs. Long-Term Effects: Deterministic vs. Stochastic
Scientists classify the health effects of ionizing radiation into two main categories:
- Deterministic Effects: These happen only after a specific threshold dose is reached, and the severity increases with the dose. Think of it like getting a sunburn – you need a certain amount of sun exposure, and more exposure means a worse burn. Examples include skin burns, hair loss, cataracts, sterility, and Acute Radiation Syndrome (ARS) following very high, short-term exposure.
- Stochastic Effects: These are chance-based effects, like cancer or potential genetic mutations passed to offspring. The probability of these effects occurring increases with dose, but the severity doesn't depend on the dose received. It's like buying lottery tickets – the more you buy (higher dose), the higher your chance of winning (developing cancer), but the prize (the cancer itself) is the same regardless of how many tickets you bought. The widely used Linear No-Threshold (LNT) model suggests that any dose carries some risk, no matter how small.
The Dose Makes the Poison
The amount (dose), type, and duration of radiation exposure are critical.
- Acute High Doses (received quickly): Can cause immediate effects like nausea, vomiting, fatigue, blood cell changes, and, at very high levels, ARS or even death. Specific thresholds exist for effects like temporary sterility, cataracts, and skin injury.
- Lower Doses / Chronic Exposure (over time): Primarily increases the long-term risk of developing cancer (like leukemia, thyroid, breast, lung cancers) years or decades later. The body can often repair damage more effectively when the dose is received slowly. Studies on atomic bomb survivors and those exposed after Chernobyl provide significant evidence for these long-term risks.
- Localized Exposure: Even if the whole-body dose isn't high, focused radiation can damage specific organs (e.g., cataracts from eye exposure, skin reddening).
Table 1: Acute Health Effects of Ionizing Radiation by Dose
Different Radiation Types, Different Behaviors
Ionizing radiation isn't monolithic. Alpha, beta, and gamma radiations differ significantly:
- Alpha (α): Heavy particles, stopped easily by skin or paper. It has a low external risk but is very damaging if inhaled or ingested (high internal hazard). Think of them as cannonballs – short range but high impact up close.
- Beta (β): Lighter electrons penetrate tissue a few centimeters. Can cause skin burns externally and are hazardous internally like bullets – moderate range and impact.
- Gamma (γ) and X-rays: These high-energy waves penetrate deeply through the body. They are a significant external hazard requiring dense shielding (like lead). Like pervasive lasers, they are long–range and capable of irradiating the whole body.
Table 2: Comparison of Alpha, Beta, and Gamma Radiation
Vulnerable Populations
Children, fetuses, and those with weakened immune systems are more sensitive to radiation. Their cells divide rapidly, making them more susceptible to DNA damage, and children have longer lifespans for cancers to potentially develop. Special care is needed, like minimizing medical radiation when possible.
Radiation in Medicine: Benefits vs. Long-Term Risks
Radiation is crucial for medical diagnosis (X-rays, CT scans) and cancer treatment (radiotherapy).
- Radiotherapy uses high doses to kill cancer cells, but depending on the treated area, it can cause long-term side effects like secondary cancers, cataracts, organ-specific issues (lung scarring, bowel changes, infertility), fatigue, and skin changes. Modern techniques aim to minimize damage to healthy tissue.
- Diagnostic Imaging: Uses much lower doses, but cumulative exposure slightly increases lifetime cancer risk. Generally, the diagnostic benefit outweighs this small risk.
Environmental and Non-Ionizing Radiation
We're constantly exposed to natural background radiation. Radon gas in homes is a key source and a known lung cancer risk. Nuclear accidents and weapons testing have also contributed to environmental radiation, providing data on high-dose effects.
Non-ionizing radiation (UV, ELF, RF) also matters:
- UV Radiation (Sunlight, Tanning Beds): The leading cause of skin cancer. It also causes sunburn and eye damage, but it is needed for Vitamin D. Sun safety is key!
- ELF-EMF (Power Lines, Appliances): High intensities can stimulate nerves. Long-term effects (e.g., cancer links) are still debated and researched.
- RF Radiation (Mobile Phones, Wi-Fi): Intense exposure causes heating. Research on long-term risks like cancer is ongoing, and results are inconsistent.
Why This Matters: The Bigger Picture
Understanding radiation's effects is vital for:
- Public Health: Setting safety limits for workers and the public.
- Medicine: Optimizing treatments and diagnostic tools while minimizing harm.
- Environmental Protection: Managing natural sources like radon and responding to accidents.
- Informed Decisions: Allowing individuals and society to weigh the risks and benefits of radiation-related technologies.
The Takeaway
Our relationship with radiation involves balancing its indispensable benefits in medicine and industry against inherent health risks tied closely to exposure type, dose, and duration. The science shows that while high doses cause definite, acute harm, even lower doses contribute to a cumulative lifetime risk, particularly for cancer.
Informed by extensive research and global health organizations, the key message is prudent avoidance through the ALARA principle—keeping exposure As Low As Reasonably Achievable. This is especially crucial for vulnerable groups like children and during pregnancy. Continuous research and careful regulation remain vital as we harness radiation's benefits while respecting and managing its potential risks in our technological world.